UTI Energy Technology: A Sustainable Future
UTI energy technology, a groundbreaking field, harnesses the power of nature to provide clean and sustainable energy solutions. This technology leverages the unique properties of various natural resources, such as […]
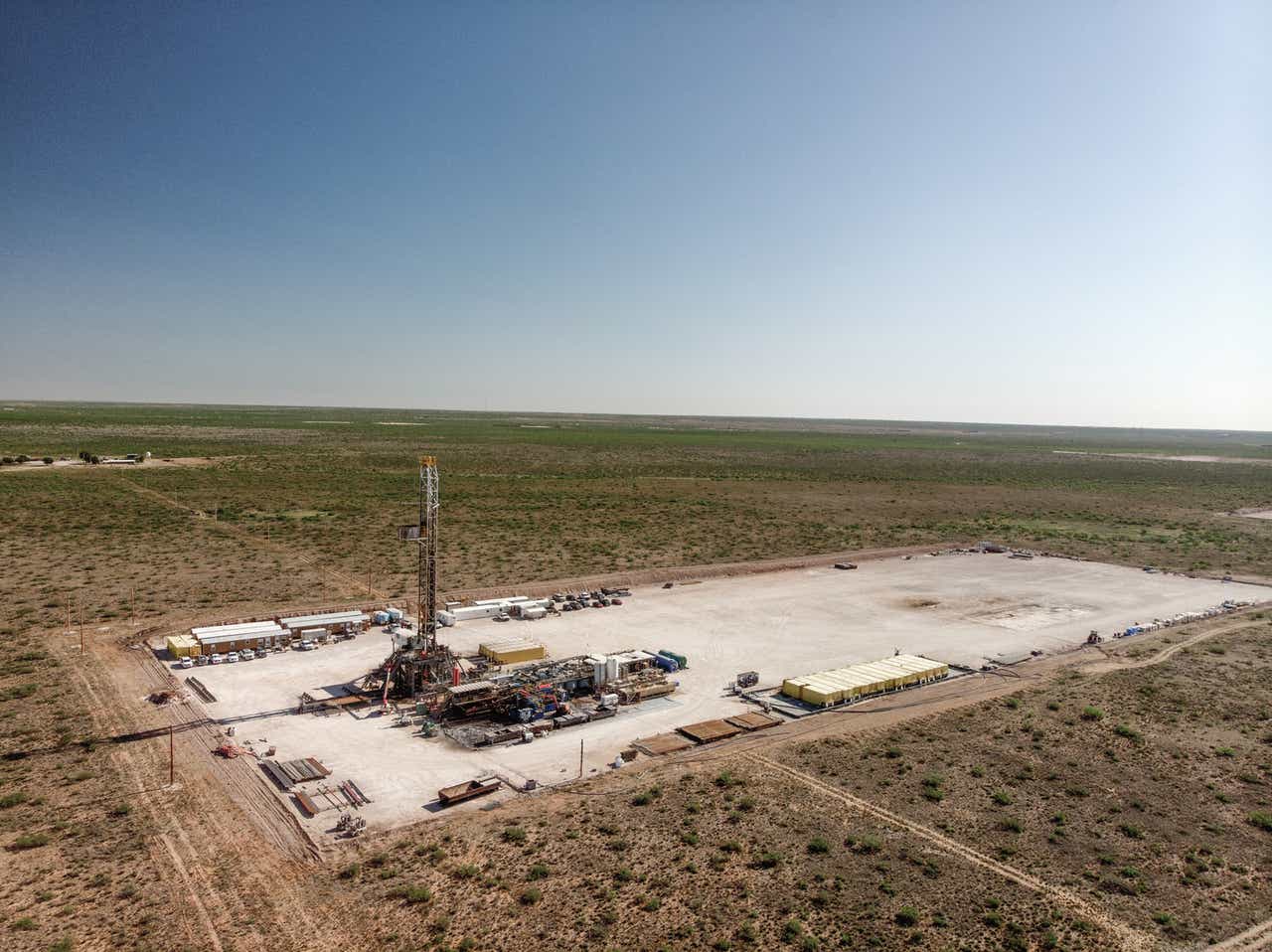
UTI energy technology, a groundbreaking field, harnesses the power of nature to provide clean and sustainable energy solutions. This technology leverages the unique properties of various natural resources, such as geothermal heat, solar radiation, and wind, to generate electricity and heat. UTI energy technologies have emerged as a crucial solution to address the pressing need for environmentally friendly energy sources, offering a viable alternative to traditional fossil fuels.
The development of UTI energy technologies has been driven by a growing awareness of the environmental impact of fossil fuel consumption. From harnessing the power of geothermal energy deep within the Earth to capturing the sun’s energy through solar panels, these technologies are transforming the energy landscape. UTI energy technologies have seen widespread adoption in various industries, including residential, commercial, and industrial sectors, demonstrating their versatility and potential for widespread impact.
Introduction to UTI Energy Technology
UTI (Ultrasonic Transmission Imaging) energy technology is a cutting-edge approach to harnessing the power of ultrasound for various applications. It involves using high-frequency sound waves to generate images and manipulate materials at the microscopic level. This technology has emerged as a promising solution for a wide range of industries, including medicine, manufacturing, and energy.
History and Evolution of UTI Energy Technology
The foundation of UTI energy technology can be traced back to the discovery of ultrasound in the 19th century. Early research focused on understanding the properties of sound waves and their interaction with matter. In the early 20th century, the development of piezoelectric materials led to the creation of devices capable of generating and detecting ultrasound. These advancements paved the way for the emergence of ultrasound imaging techniques, primarily in the medical field.
Over the years, UTI energy technology has evolved significantly, driven by advancements in materials science, electronics, and computing power. The development of more powerful ultrasound transducers, sophisticated signal processing algorithms, and high-resolution imaging systems has enabled the use of ultrasound for a broader range of applications.
Real-World Applications of UTI Energy Technology
UTI energy technology has found numerous applications across various industries, demonstrating its versatility and potential.
Medical Applications
UTI energy technology plays a crucial role in medical diagnostics and treatment.
- Ultrasound imaging is widely used for non-invasive visualization of internal organs and tissues, providing valuable information for diagnosis and monitoring of various conditions.
- Focused ultrasound therapy utilizes high-intensity ultrasound waves to target and destroy diseased tissue, offering a minimally invasive treatment option for conditions like cancer and neurological disorders.
- Ultrasound-guided surgery enhances precision and accuracy during surgical procedures, minimizing damage to surrounding healthy tissue.
Manufacturing Applications
UTI energy technology has revolutionized manufacturing processes, enabling precise control and manipulation of materials at the microscopic level.
- Ultrasonic welding is a highly efficient and reliable method for joining dissimilar materials, widely used in the automotive, electronics, and aerospace industries.
- Ultrasonic cleaning utilizes high-frequency sound waves to remove contaminants from surfaces, improving product quality and reducing manufacturing costs.
- Ultrasonic machining is a precise and efficient method for cutting and shaping hard materials, used in the production of tools, dies, and medical implants.
Energy Applications
UTI energy technology is gaining momentum in the energy sector, offering innovative solutions for energy generation, storage, and transmission.
- Ultrasonic energy harvesting converts mechanical vibrations into electrical energy, providing a sustainable source of power for wireless sensors and other devices.
- Ultrasonic cavitation enhances the efficiency of fuel cells by increasing the surface area of electrodes and promoting chemical reactions.
- Ultrasonic cleaning of solar panels removes dirt and debris, improving their efficiency and extending their lifespan.
Types of UTI Energy Technologies
UTI energy technologies, also known as unconventional thermal insulation (UTI) technologies, represent a diverse range of approaches to minimize heat loss and improve energy efficiency. These technologies deviate from conventional insulation methods and utilize innovative materials and techniques to achieve superior thermal performance.
Types of UTI Energy Technologies
UTI energy technologies can be broadly categorized based on their principles and applications. The following are some of the most common types:
- Vacuum Insulation Panels (VIPs): VIPs consist of a core material, such as silica aerogel or expanded perlite, enclosed within a vacuum-sealed envelope. The vacuum eliminates air molecules, which are the primary carriers of heat transfer by conduction and convection. This creates a high thermal resistance, significantly reducing heat loss. VIPs are commonly used in building insulation, refrigeration, and cryogenic applications.
- Aerogel Insulation: Aerogels are lightweight, porous materials with extremely low thermal conductivity. They are composed of a network of interconnected nanoparticles that trap air, creating a highly insulating material. Aerogels are often used in building insulation, aerospace applications, and high-temperature insulation.
- Phase Change Materials (PCMs): PCMs are materials that absorb and release heat during phase transitions, such as melting or freezing. They act as thermal energy storage devices, absorbing heat during the day and releasing it at night, thus moderating temperature fluctuations. PCMs are used in building insulation, solar energy storage, and textile applications.
- Reflective Insulation: Reflective insulation utilizes highly reflective materials, such as aluminum foil or metallic coatings, to reduce radiative heat transfer. The reflective surfaces minimize heat absorption and re-radiation, effectively reducing heat gain or loss. Reflective insulation is commonly used in attics, roofs, and walls.
- Dynamic Insulation: Dynamic insulation involves actively controlling the thermal properties of insulation materials. This can be achieved through various techniques, such as adjusting the thickness of the insulation layer, using smart materials that respond to temperature changes, or employing vacuum-based insulation systems. Dynamic insulation allows for optimized thermal performance based on real-time conditions.
Environmental Impact of UTI Energy Technology
UTI energy technology, while promising in terms of providing a sustainable energy source, also comes with potential environmental impacts that need careful consideration. This section will explore the benefits and drawbacks of UTI energy technology in relation to its environmental impact, analyzing its potential influence on greenhouse gas emissions and climate change, and identifying potential risks and challenges associated with its sustainability.
Greenhouse Gas Emissions and Climate Change
The potential impact of UTI energy technology on greenhouse gas emissions and climate change is a critical area of research. While UTI energy technologies aim to reduce reliance on fossil fuels, their impact on climate change can vary depending on the specific technology and its implementation.
- Some UTI energy technologies, like solar and wind power, have a minimal impact on greenhouse gas emissions throughout their lifecycle. This is because they do not produce emissions during operation. However, the manufacturing and disposal of these technologies can generate some emissions.
- Other UTI energy technologies, such as biomass energy, can have a more complex impact on greenhouse gas emissions. While burning biomass can release carbon dioxide, it is considered carbon-neutral if the biomass is grown sustainably and replaces fossil fuels. However, if the biomass is grown on land that was previously used for carbon sequestration, the overall impact on climate change can be negative.
- UTI energy technologies like geothermal energy can have a minimal impact on greenhouse gas emissions during operation. However, the construction of geothermal power plants can result in the release of greenhouse gases, especially if they involve drilling into deep geological formations.
The potential impact of UTI energy technology on climate change is further complicated by the fact that the technology is still in its early stages of development. As the technology matures, it is expected that the environmental impact will become more defined and predictable. However, it is crucial to ensure that the development and deployment of UTI energy technologies are conducted in a way that minimizes their environmental impact and contributes to a sustainable future.
Economic and Social Implications of UTI Energy Technology
The development and deployment of UTI energy technology hold significant implications for the global economy and society. Understanding the economic feasibility, cost-effectiveness, and potential social impacts of this technology is crucial for informed decision-making and successful implementation.
Economic Feasibility and Cost-Effectiveness
The economic feasibility of UTI energy technology is a complex issue that depends on various factors, including the cost of development, production, and deployment, as well as the potential revenue streams generated by the technology. While the initial costs of developing and deploying UTI energy technology may be high, the long-term benefits, such as reduced reliance on fossil fuels and lower energy costs, could outweigh the initial investment.
- Cost of Development and Deployment: The development and deployment of UTI energy technology require significant investment in research, development, and infrastructure. The cost of developing UTI energy technologies can vary depending on the specific technology and the scale of deployment. For example, developing and deploying large-scale UTI energy plants would require significant capital investment.
- Cost-Effectiveness: The cost-effectiveness of UTI energy technology is determined by comparing the costs of producing energy from UTI sources with the costs of producing energy from traditional sources, such as fossil fuels. The cost of producing energy from UTI sources is generally lower than the cost of producing energy from fossil fuels. However, the cost-effectiveness of UTI energy technology can vary depending on the specific technology, the location, and the availability of resources.
- Revenue Streams: UTI energy technologies can generate revenue through the sale of electricity, heat, and other energy products. The revenue generated from UTI energy technologies can be used to offset the costs of development, production, and deployment.
Social Impacts of UTI Energy Technology
The social impacts of UTI energy technology are multifaceted and can affect employment, infrastructure, and communities. It is essential to consider these impacts during the development and deployment of this technology.
- Employment: The development and deployment of UTI energy technology can create new jobs in the energy sector. These jobs include research and development, manufacturing, construction, operation, and maintenance.
- Infrastructure: UTI energy technology can require significant investments in infrastructure, such as power grids, pipelines, and storage facilities. These investments can stimulate economic growth and create jobs in construction and engineering sectors.
- Communities: The deployment of UTI energy technology can have significant impacts on communities, both positive and negative. For example, the development of UTI energy plants can lead to increased economic activity in local communities. However, it is essential to ensure that the development and deployment of UTI energy technology are done in a way that minimizes negative impacts on communities, such as environmental pollution and displacement.
Role of Government Policies and Regulations
Government policies and regulations play a crucial role in promoting the adoption of UTI energy technology. These policies can include incentives, subsidies, and regulations that encourage the development and deployment of UTI energy technologies.
- Incentives and Subsidies: Governments can provide incentives and subsidies to encourage the development and deployment of UTI energy technologies. These incentives can include tax breaks, grants, and loan guarantees.
- Regulations: Governments can implement regulations that promote the use of UTI energy technologies. These regulations can include renewable energy standards, carbon pricing mechanisms, and regulations that promote the development of UTI energy infrastructure.
Future Trends and Developments in UTI Energy Technology
The field of UTI energy technology is constantly evolving, driven by advancements in materials science, nanotechnology, and energy storage. These developments hold the potential to revolutionize the way we generate, store, and utilize energy, paving the way for a more sustainable and efficient energy future.
Emerging Trends and Innovations in UTI Energy Technology
The ongoing research and development in UTI energy technology are focusing on several key areas.
- Enhanced Energy Storage Capacity: Researchers are exploring new materials and designs for UTI devices to increase their energy storage capacity. This involves developing advanced electrode materials with higher surface areas, improved conductivity, and enhanced electrochemical stability.
- Improved Efficiency and Durability: Efforts are underway to enhance the efficiency and durability of UTI devices. This includes optimizing the electrolyte composition, electrode structure, and device fabrication processes to minimize energy loss and extend the lifespan of the devices.
- Miniaturization and Integration: There is a growing interest in miniaturizing UTI devices to enable their integration into various applications, such as wearable electronics, micro-sensors, and implantable medical devices.
- Hybrid and Multifunctional Systems: Research is exploring the development of hybrid UTI systems that combine different energy storage mechanisms to achieve higher energy density, power density, and improved performance.
Potential Future Applications and Advancements in UTI Energy Technology
UTI energy technology is poised to play a crucial role in addressing the growing global energy demands and environmental concerns.
- Renewable Energy Integration: UTI devices can be used to store energy generated from renewable sources, such as solar and wind power, providing a reliable and efficient way to manage intermittent energy supply.
- Electric Vehicles: UTI energy technology has the potential to revolutionize electric vehicles by enabling the development of lighter, more powerful, and longer-lasting batteries.
- Grid-Scale Energy Storage: UTI devices can be integrated into the power grid to enhance grid stability, reduce peak demand, and improve the reliability of power supply.
- Portable Electronics: UTI technology can enable the development of smaller, lighter, and more powerful batteries for portable electronic devices, such as smartphones, laptops, and tablets.
- Medical Devices: UTI devices have the potential to power implantable medical devices, such as pacemakers, insulin pumps, and cochlear implants, providing a long-lasting and reliable energy source.
Research Areas and Challenges that Need to be Addressed for Further Development and Adoption of UTI Energy Technology
Despite the significant potential of UTI energy technology, there are still several research challenges that need to be addressed before its widespread adoption.
- Cost Reduction: The high cost of materials and manufacturing processes is a major barrier to the widespread adoption of UTI devices. Research is ongoing to develop cost-effective materials and fabrication methods to make UTI technology more affordable.
- Safety and Environmental Concerns: The safety and environmental impact of UTI devices need to be carefully evaluated. Research is focused on developing safer electrolytes and electrode materials that do not pose environmental risks.
- Scalability and Manufacturing: Scaling up the production of UTI devices to meet the growing demand requires efficient and cost-effective manufacturing processes. Research is being conducted to develop scalable and sustainable manufacturing techniques.
- Standardization and Interoperability: The lack of standardized testing protocols and interoperability between different UTI devices can hinder their adoption. Research is needed to develop standardized testing procedures and ensure compatibility between different UTI systems.
Case Studies and Examples: Uti Energy Technology
To understand the real-world impact of UTI energy technologies, it’s essential to examine practical applications. This section explores various case studies, highlighting the technologies used, their environmental and social benefits, and the challenges faced during implementation.
Case Studies of UTI Energy Technologies, Uti energy technology
The following table provides a snapshot of different UTI energy technology applications, their impact, and challenges:
Application | Technology | Impact | Challenges |
---|---|---|---|
Waste-to-Energy Plant in Copenhagen, Denmark | Plasma Gasification | Converts municipal solid waste into electricity and heat, reducing landfill reliance and greenhouse gas emissions. | High initial investment costs, public concerns about potential emissions, and complex waste sorting requirements. |
Geothermal Power Plant in Iceland | Geothermal Energy | Provides clean and sustainable electricity, reducing reliance on fossil fuels and promoting energy independence. | Limited geographical availability, potential for seismic activity, and environmental concerns related to groundwater contamination. |
Solar Thermal Power Plant in Spain | Concentrated Solar Power (CSP) | Generates electricity using solar energy, reducing carbon emissions and promoting renewable energy sources. | High land requirements, intermittent nature of solar energy, and challenges associated with storage and grid integration. |
Tidal Power Plant in France | Tidal Energy | Harnesses the kinetic energy of ocean tides to generate electricity, providing a reliable and predictable source of renewable energy. | Limited geographic availability, potential impact on marine ecosystems, and high construction costs. |
Closure
As we navigate the challenges of climate change and the pursuit of sustainable energy solutions, UTI energy technologies offer a beacon of hope. These technologies are poised to play a pivotal role in shaping the future of energy, contributing to a cleaner, more sustainable world. With ongoing research and development, UTI energy technologies are constantly evolving, promising even greater efficiency and affordability in the years to come.
UTI Energy Technology is at the forefront of innovation, developing cutting-edge solutions for the energy sector. Their commitment to sustainability is evident in their partnerships with companies like pinteng technology limited , who share a similar dedication to environmental responsibility.
By collaborating with such forward-thinking partners, UTI Energy Technology continues to push the boundaries of energy efficiency and create a more sustainable future.