Fiber Technologies: Revolutionizing Communication
Fiber technologies have revolutionized the way we communicate and access information, ushering in a new era of high-speed data transmission. This groundbreaking technology, utilizing thin strands of glass or plastic […]
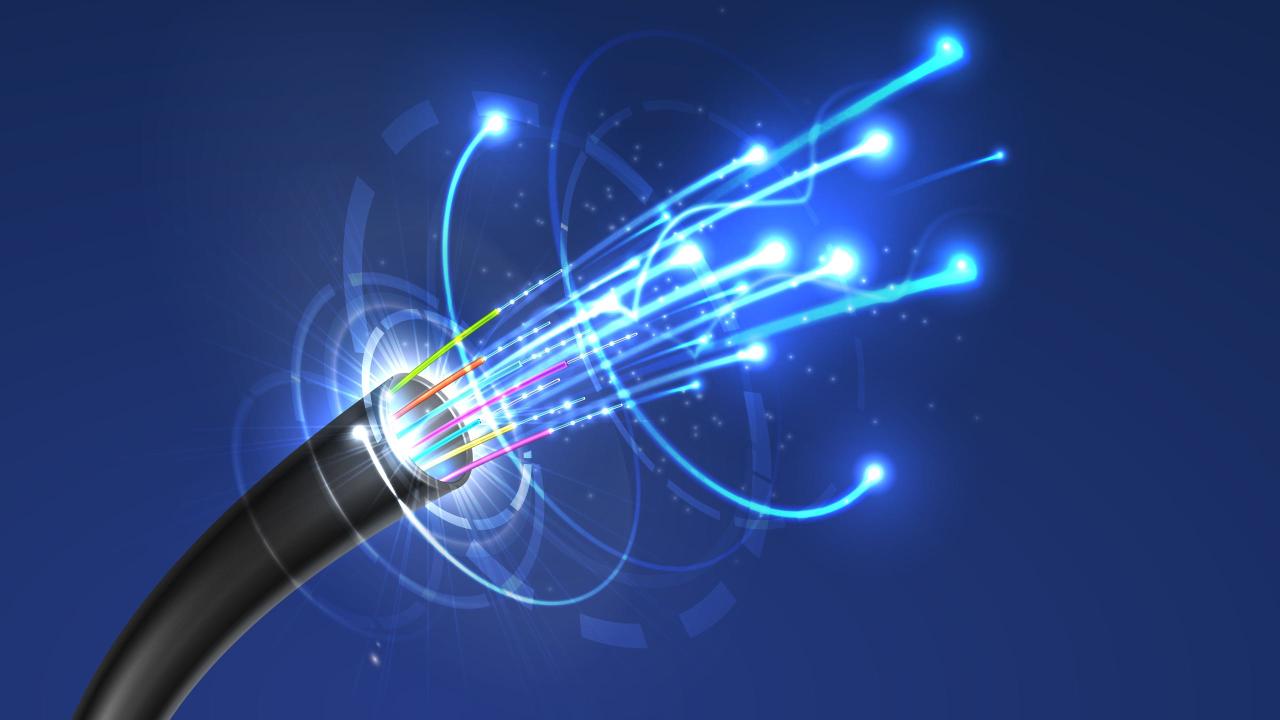
Fiber technologies have revolutionized the way we communicate and access information, ushering in a new era of high-speed data transmission. This groundbreaking technology, utilizing thin strands of glass or plastic to transmit light pulses, has transformed industries, enabling faster, more reliable connectivity than ever before.
From the early days of fiber optic cables to the advanced networks of today, fiber technologies have continually evolved, pushing the boundaries of data transfer. The fundamental principle of light transmission through optical fibers, a fascinating interplay of physics and engineering, has opened up a world of possibilities, enabling us to connect, share, and innovate like never before.
Introduction to Fiber Technologies
Fiber technologies, particularly fiber optic technology, have revolutionized modern communication and data transmission. They form the backbone of high-speed internet, telecommunications networks, and various other applications that rely on rapid and reliable data transfer.
Fiber optic technology utilizes the transmission of light pulses through thin strands of glass or plastic called optical fibers. This method offers significant advantages over traditional copper-based systems, enabling faster data rates, greater bandwidth, and improved signal integrity.
History of Fiber Optic Technology Development
The development of fiber optic technology has been a gradual process, starting with the early exploration of light propagation through glass fibers.
- 1950s: Early research focused on the use of glass fibers for image transmission, laying the foundation for later developments in communication.
- 1960s: The invention of the laser and the development of low-loss optical fibers paved the way for practical applications of fiber optic communication.
- 1970s: The first commercial fiber optic systems were deployed, primarily for long-distance telecommunications.
- 1980s: Advancements in fiber optic technology led to the widespread adoption of fiber optic cables for various applications, including cable television and data networks.
- 1990s and beyond: Continued research and development have led to the emergence of high-capacity fiber optic networks, enabling the transmission of vast amounts of data at unprecedented speeds.
Fundamental Principles of Light Transmission Through Optical Fibers
Light transmission through optical fibers relies on the principle of total internal reflection.
An optical fiber consists of a core made of glass or plastic with a higher refractive index than the surrounding cladding. When light enters the core at a specific angle, it undergoes total internal reflection, bouncing back and forth within the core. This process allows light to travel long distances through the fiber without significant loss of signal strength.
The refractive index of a material determines how much light bends when it passes from one medium to another. The core of an optical fiber has a higher refractive index than the cladding, causing light to bend towards the core when it reaches the boundary between the two. This bending effect, combined with the angle of incidence, leads to total internal reflection.
Types of Fiber Optic Cables
Fiber optic cables are the backbone of modern communication networks, enabling high-speed data transmission over long distances with minimal signal degradation. Understanding the different types of fiber optic cables is crucial for selecting the right cable for specific applications.
Fiber Optic Cable Classification
Fiber optic cables can be classified based on several factors, including core diameter, cladding material, and mode of transmission. These factors influence the cable’s performance characteristics, such as bandwidth, signal attenuation, and cost.
Core Diameter
The core diameter refers to the thickness of the central glass fiber that carries the light signal. Fiber optic cables are broadly categorized into two main types based on their core diameter: single-mode and multimode fibers.
Cladding Material
The cladding material surrounds the core and is typically made of glass with a lower refractive index than the core. This difference in refractive index ensures that light is reflected back into the core, preventing signal loss.
Mode of Transmission
The mode of transmission refers to the way light travels through the fiber. Single-mode fibers allow only one mode of light to propagate, while multimode fibers allow multiple modes to travel simultaneously.
Single-Mode Fiber
Single-mode fibers have a small core diameter (typically 8.3µm or 9µm) that allows only one mode of light to propagate. This results in a highly focused beam of light with minimal dispersion, enabling long-distance transmission with high bandwidth and low signal attenuation.
Characteristics of Single-Mode Fiber
- Small core diameter: 8.3µm or 9µm.
- High bandwidth: Supports high data rates, exceeding 100 Gbps.
- Low attenuation: Minimal signal loss over long distances.
- Long reach: Can transmit signals over distances exceeding 100 km.
- High cost: More expensive than multimode fibers.
Applications of Single-Mode Fiber
Single-mode fibers are widely used in high-performance applications, including:
- Long-haul telecommunications: Connecting cities and countries.
- Data centers: Providing high-speed connectivity between servers and storage devices.
- Fiber-to-the-home (FTTH): Delivering high-speed internet to residential customers.
- Scientific research: Conducting experiments requiring high-precision measurements.
Multimode Fiber
Multimode fibers have a larger core diameter (typically 50µm or 62.5µm) than single-mode fibers, allowing multiple modes of light to propagate simultaneously. This results in a wider beam of light with higher dispersion, limiting the transmission distance and bandwidth compared to single-mode fibers.
Characteristics of Multimode Fiber
- Large core diameter: 50µm or 62.5µm.
- Lower bandwidth: Supports lower data rates compared to single-mode fibers.
- Higher attenuation: More signal loss over long distances.
- Shorter reach: Typically used for shorter distances, up to a few kilometers.
- Lower cost: Less expensive than single-mode fibers.
Applications of Multimode Fiber
Multimode fibers are commonly used in shorter-distance applications, such as:
- Local area networks (LANs): Connecting computers and devices within a building or campus.
- Industrial automation: Monitoring and controlling machinery and processes.
- Medical imaging: Transmitting data from medical equipment to display devices.
- Building infrastructure: Providing connectivity for security systems, lighting control, and other building services.
Fiber Optic Cable Construction: Fiber Technologies
Fiber optic cables are the backbone of modern communication networks, transmitting data at incredibly high speeds. Understanding the construction of these cables is crucial for efficient installation, maintenance, and troubleshooting. This section will delve into the different components that make up a fiber optic cable, explore the types of connectors used, and discuss the importance of proper termination and splicing techniques.
Components of a Fiber Optic Cable
The construction of a fiber optic cable is designed to protect the delicate optical fiber from damage and ensure reliable signal transmission. It consists of several layers, each serving a specific purpose:
- Core: This is the heart of the fiber optic cable, made of highly purified glass or plastic. It carries the light pulses that transmit data. The core’s diameter, typically measured in micrometers, determines the fiber’s capacity and transmission characteristics.
- Cladding: Surrounding the core, the cladding is another layer of glass or plastic with a lower refractive index than the core. This difference in refractive index causes light to reflect internally within the core, minimizing signal loss.
- Buffer: The buffer is a protective layer that surrounds the core and cladding. It’s usually made of a resilient plastic material that safeguards the fiber from abrasion, moisture, and other environmental hazards.
- Jacket: The outermost layer of the cable, the jacket provides further protection and strength. It can be made from different materials like PVC, polyethylene, or a combination of materials, depending on the cable’s intended application and environmental conditions.
Fiber Optic Connectors
Connectors are crucial components that enable the connection of fiber optic cables to equipment like routers, switches, and transceivers. They provide a secure and reliable interface for light transmission. Here are some common types of fiber optic connectors:
- ST (Straight Tip): One of the earliest types of connectors, ST connectors feature a bayonet-style locking mechanism. They are known for their ruggedness and are often used in telecommunications and industrial applications.
- SC (Subscriber Connector): SC connectors utilize a push-pull mechanism for easy connection and disconnection. They are widely used in telecommunications and data centers due to their reliability and ease of use.
- FC (Fiber Connector): FC connectors feature a threaded locking mechanism that provides a secure connection. They are commonly used in high-performance applications where stability is critical, such as in laboratory equipment and long-haul fiber optic links.
- LC (Lucent Connector): LC connectors are small and compact, offering high density in applications where space is limited. They are becoming increasingly popular in data centers and high-speed networking.
- MT-RJ (Mechanical Transfer Registered Jack): MT-RJ connectors are also known as “mini-SC” connectors due to their similarity to SC connectors. They are commonly used in multi-fiber applications and are gaining popularity in fiber-to-the-home (FTTH) networks.
Cable Termination and Splicing Techniques
Proper termination and splicing techniques are essential for maintaining signal integrity and ensuring reliable data transmission.
Cable Termination
Cable termination involves preparing the end of a fiber optic cable for connection to a connector. This process typically involves the following steps:
- Stripping: The outer jacket and buffer layers are carefully removed to expose the fiber optic core.
- Cleaning: The exposed fiber is cleaned to remove any contaminants that could interfere with signal transmission.
- Cleaving: The fiber is cleaved to create a perfectly smooth and perpendicular end face.
- Connector Insertion: The prepared fiber is inserted into the connector, ensuring proper alignment and a secure connection.
Splicing
Splicing is used to join two fiber optic cables together, creating a continuous link. This process requires specialized equipment and skilled technicians to ensure proper alignment and minimize signal loss.
- Fusion Splicing: This method uses heat to melt the ends of two fibers together, creating a permanent and high-quality splice. Fusion splicing is widely used in telecommunications and data centers due to its low signal loss and durability.
- Mechanical Splicing: This method uses mechanical components to align and hold the fibers together. Mechanical splicing is a faster and less expensive option than fusion splicing, but it typically results in higher signal loss.
Fiber Optic Transmission Systems
Fiber optic transmission systems leverage the unique properties of light to transmit data over long distances with minimal signal degradation. This technology has revolutionized communication networks, enabling high-speed data transfer and improved signal quality.
Principles of Optical Signal Transmission
Optical signal transmission through fiber optic cables relies on the principle of total internal reflection. Light travels through the core of the fiber, a transparent material with a higher refractive index than the cladding surrounding it. When light strikes the boundary between the core and cladding at an angle greater than the critical angle, it is reflected back into the core. This phenomenon ensures that the light remains confined within the fiber, allowing for efficient signal propagation over long distances.
Components of a Fiber Optic Transmission System
A typical fiber optic transmission system comprises three essential components:
Transmitter
The transmitter converts electrical signals into optical signals. It consists of a light source, such as a laser diode or LED, which emits light at a specific wavelength. The electrical signal modulates the intensity of the light, encoding data onto the optical signal.
Receiver
The receiver converts the optical signal back into an electrical signal. It consists of a photodetector, which converts the light into an electrical current, and an amplifier to boost the signal strength.
Optical Amplifier
Optical amplifiers amplify the optical signal directly without converting it to an electrical signal. They are used to compensate for signal loss over long distances, allowing for the transmission of data over hundreds or even thousands of kilometers.
Modulation Techniques
Fiber optic communication employs various modulation techniques to encode data onto the optical signal. These techniques alter the properties of the light wave, such as intensity, frequency, or phase, to represent different data bits.
Intensity Modulation (IM)
Intensity modulation (IM) is the simplest and most common modulation technique. It varies the intensity of the light wave to represent data bits. A higher intensity represents a “1” bit, while a lower intensity represents a “0” bit.
Frequency Modulation (FM)
Frequency modulation (FM) varies the frequency of the light wave to encode data. Different frequencies correspond to different data bits. This technique is less susceptible to noise and interference than IM, but it requires more complex equipment.
Phase Modulation (PM)
Phase modulation (PM) changes the phase of the light wave to represent data bits. Different phases correspond to different data bits. This technique offers high bandwidth efficiency and is used in high-speed fiber optic communication systems.
Future Trends in Fiber Technologies
Fiber optic technology has been a cornerstone of modern communication, and its evolution continues to drive innovation and push the boundaries of what’s possible. The future of fiber optics is bright, with exciting advancements in transmission speeds, network capacity, and innovative architectures poised to reshape the digital landscape.
Higher Transmission Speeds and Increased Capacity
The demand for higher bandwidth is relentless, fueled by the increasing adoption of data-intensive applications like streaming services, cloud computing, and the Internet of Things (IoT). Fiber optic technology is at the forefront of meeting this demand, with ongoing advancements in transmission speeds and network capacity.
- Multi-level Modulation: This technique increases the amount of data that can be transmitted over a single fiber by using multiple signal levels. This allows for higher data rates and more efficient use of the available bandwidth.
- Space Division Multiplexing (SDM): This technique involves using multiple optical paths within a single fiber to increase the overall capacity. It is particularly beneficial for long-haul networks where the need for higher capacity is paramount.
- Fiber to the Home (FTTH): FTTH deployments are expanding rapidly, bringing high-speed internet access to homes and businesses. This is driving the demand for fiber optic cables with higher bandwidth capabilities to support the increasing data demands of residential users.
Optical Switching and Wavelength Division Multiplexing (WDM)
Optical switching and WDM are crucial technologies for enabling high-capacity, flexible, and scalable fiber optic networks.
Optical Switching
Optical switching allows for the direct routing of optical signals without the need for electronic conversion, reducing latency and improving network efficiency.
- Optical Cross-Connects (OXCs): These devices provide a flexible and dynamic way to route optical signals, enabling efficient network management and service provisioning.
- Optical Add-Drop Multiplexers (OADMs): OADMs allow for the selective addition or removal of optical channels from a fiber, enabling the efficient use of bandwidth and the creation of flexible network architectures.
Wavelength Division Multiplexing (WDM)
WDM technology enables the transmission of multiple optical signals over a single fiber by using different wavelengths. This allows for a significant increase in network capacity and efficiency.
- Dense Wavelength Division Multiplexing (DWDM): DWDM systems utilize a large number of closely spaced wavelengths, enabling the transmission of massive amounts of data over a single fiber. For instance, a single DWDM fiber can carry data equivalent to hundreds of thousands of traditional copper telephone lines.
- Coherent Optical Communication: Coherent optical communication systems use advanced signal processing techniques to enhance the performance of WDM systems. This allows for higher data rates, longer transmission distances, and improved signal quality. Coherent systems are particularly well-suited for long-haul applications and submarine cable networks.
Advanced Network Architectures, Fiber technologies
Fiber optic technology is driving the development of advanced network architectures that are more efficient, scalable, and resilient.
- Software-Defined Networking (SDN): SDN allows for the centralized control and management of network resources, enabling dynamic provisioning, optimization, and automation. Fiber optic networks are well-suited for SDN deployments due to their high bandwidth and low latency.
- Network Function Virtualization (NFV): NFV enables the virtualization of network functions, allowing for greater flexibility, agility, and cost-effectiveness. Fiber optic networks provide the high bandwidth and low latency required for efficient NFV deployments.
- Cloud-based Networking: The rise of cloud computing is driving the demand for high-bandwidth, low-latency connectivity. Fiber optic networks are playing a critical role in connecting data centers and cloud platforms, enabling the seamless delivery of cloud services.
Final Conclusion
As we delve deeper into the future of fiber technologies, the potential for even greater advancements is undeniable. With the emergence of new technologies like optical switching, wavelength division multiplexing (WDM), and coherent optical communication, the landscape of data transmission is poised for another dramatic transformation. Fiber technologies will continue to shape our digital world, enabling faster speeds, increased capacity, and seamless connectivity, ultimately connecting us in ways we can only begin to imagine.
Fiber technologies have revolutionized communication and data transfer, but they also pose challenges for cleaning and maintenance. Specialized cleaning solutions, like franklin cleaning technology , are crucial for ensuring optimal performance and longevity of these delicate fiber optic components.
By addressing the specific needs of fiber optics, these cleaning technologies contribute to the reliability and efficiency of our increasingly interconnected world.